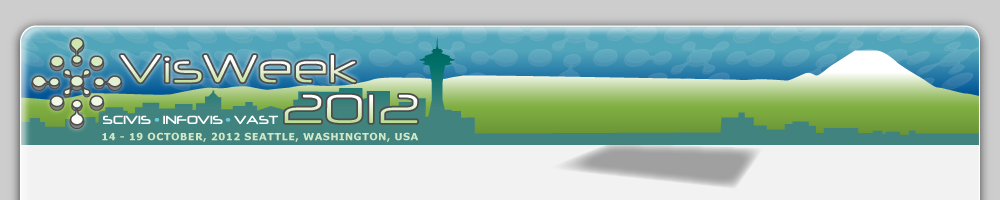
Color mapping and semitransparent layering play an important role in many visualization scenarios, such as information visualization and volume rendering. The combination of color and transparency is still dominated by standard alpha-compositing using the Porter-Duff over operator which can result in false colors with deceiving impact on the visualization. Other more advanced methods have also been proposed, but the problem is still far from being solved. Here we present an alternative to these existing methods specifically devised to avoid false colors and preserve visual depth ordering. Our approach is data driven and follows the recently formulated knowledge-assisted visualization (KAV) paradigm. Preference data, that have been gathered in web-based user surveys, are used to train a support-vector machine model for automatically predicting an optimized hue-preserving blending. We have applied the resulting model to both volume rendering and a specific information visualization technique, illustrative parallel coordinate plots. Comparative renderings show a significant improvement over previous approaches in the sense that false colors are completely removed and important properties such as depth ordering and blending vividness are better preserved. Due to the generality of the defined data-driven blending operator, it can be easily integrated also into other visualization frameworks.
Topological techniques have proven highly successful in analyzing and visualizing scientific data. As a result, significant efforts have been made to compute structures like the Morse-Smale complex as robustly and efficiently as possible. However, the resulting algorithms, while topologically consistent, often produce incorrect connectivity as well as poor geometry. These problems may compromise or even invalidate any subsequent analysis. Moreover, such techniques may fail to improve even when the resolution of the domain mesh is increased, thus producing potentially incorrect results even for highly resolved functions. To address these problems we introduce two new algorithms: (i) a randomized algorithm to compute the discrete gradient of a scalar field that converges under refinement; and (ii) a deterministic variant which directly computes accurate geometry and thus correct connectivity of the MS complex. The first algorithm converges in the sense that on average it produces the correct result and its standard deviation approaches zero with increasing mesh resolution. The second algorithm uses two ordered traversals of the function to integrate the probabilities of the first to extract correct (near optimal) geometry and connectivity. We present an extensive empirical study using both synthetic and real-world data and demonstrates the advantages of our algorithms in comparison with several popular approaches.
This paper presents a visualization approach for detecting and exploring similarity in the temporal variation of field data. We provide an interactive technique for extracting correlations from similarity matrices which capture temporal similarity of univariate functions. We make use of the concept to extract periodic and quasiperiodic behavior at single (spatial) points as well as similarity between different locations within a field and also between different data sets. The obtained correlations are utilized for visual exploration of both temporal and spatial relationships in terms of temporal similarity. Our entire pipeline offers visual interaction and inspection, allowing for the flexibility that in particular time-dependent data analysis techniques require. We demonstrate the utility and versatility of our approach by applying our implementation to data from both simulation and measurement.
In nuclear science, density functional theory (DFT) is a powerful tool to model the complex interactions within the atomic nucleus, and is the primary theoretical approach used by physicists seeking a better understanding of fission. However DFT simulations result in complex multivariate datasets in which it is difficult to locate the crucial scission point at which one nucleus fragments into two, and to identify the precursors to scission. The Joint Contour Net (JCN) has recently been proposed as a new data structure for the topological analysis of multivariate scalar fields, analogous to the contour tree for univariate fields. This paper reports the analysis of DFT simulations using the JCN, the first application of the JCN technique to real data. It makes three contributions to visualization: (i) a set of practical methods for visualizing the JCN, (ii) new insight into the detection of nuclear scission, and (iii) an analysis of aesthetic criteria to drive further work on representing the JCN.
One potential solution to reduce the concentration of carbon dioxide in the atmosphere is the geologic storage of captured CO2 in underground rock formations, also known as carbon sequestration. There is ongoing research to guarantee that this process is both efficient and safe. We describe tools that provide measurements of media porosity, and permeability estimates, including visualization of pore structures. Existing standard algorithms make limited use of geometric information in calculating permeability of complex microstructures. This quantity is important for the analysis of biomineralization, a subsurface process that can affect physical properties of porous media. This paper introduces geometric and topological descriptors that enhance the estimation of material permeability. Our analysis framework includes the processing of experimental data, segmentation, and feature extraction and making novel use of multiscale topological analysis to quantify maximum flow through porous networks. We illustrate our results using synchrotron-based X-ray computed microtomography of glass beads during biomineralization. We also benchmark the proposed algorithms using simulated data sets modeling jammed packed bead beds of a monodispersive material.
We present a combinatorial algorithm for the general topological simplification of scalar fields on surfaces. Given a scalar field f, our algorithm generates a simplified field g that provably admits only critical points from a constrained subset of the singularities of f, while guaranteeing a small distance jjf _ gjj1 for data-fitting purpose. In contrast to previous algorithms, our approach is oblivious to the strategy used for selecting features of interest and allows critical points to be removed arbitrarily. When topological persistence is used to select the features of interest, our algorithm produces a standard _-simplification. Our approach is based on a new iterative algorithm for the constrained reconstruction of sub- and sur-level sets. Extensive experiments show that the number of iterations required for our algorithm to converge is rarely greater than 2 and never greater than 5, yielding O(n log(n)) practical time performances. The algorithm handles triangulated surfaces with or without boundary and is robust to the presence of multi-saddles in the input. It is simple to implement, fast in practice and more general than previous techniques. Practically, our approach allows a user to arbitrarily simplify the topology of an input function and robustly generate the corresponding simplified function. An appealing application area of our algorithm is in scalar field design since it enables, without any threshold parameter, the robust pruning of topological noise as selected by the user. This is needed for example to get rid of inaccuracies introduced by numerical solvers, thereby providing topological guarantees needed for certified geometry processing. Experiments show this ability to eliminate numerical noise as well as validate the time efficiency and accuracy of our algorithm. We provide a lightweight C++ implementation as supplemental material that can be used for topological cleaning on surface meshes.
Scientists, engineers and physicians are used to analyze 3D data with slice-based visualizations. Radiologists for example are trained to read slices of medical imaging data. Despite the numerous examples of sophisticated 3D rendering techniques, domain experts, who still prefer slice-based visualization do not consider these to be very useful. Since 3D renderings have the advantage of providing an overview at a glance, while 2D depictions better serve detailed analyses, it is of general interest to better combine these methods. Recently there have been attempts to bridge this gap between 2D and 3D renderings. These attempts include specialized techniques for volume picking in medical imaging data that result in repositioning slices. In this paper, we present a new volume picking technique called WYSIWYP (what you see is what you pick) that, in contrast to previous work, does not require pre-segmented data or metadata and thus is more generally applicable. The positions picked by our method are solely based on the data itself, the transfer function, and the way the volumetric rendering is perceived by the user. To demonstrate the utility of the proposed method, we apply it to automated positioning of slices in volumetric scalar fields from various application areas. Finally, we present results of a user study in which 3D locations selected by users are compared to those resulting from WYSIWYP. The user study confirms our claim that the resulting positions correlate well with those perceived by the user.
Data selection is a fundamental task in visualization because it serves as a pre-requisite to many follow-up interactions. Efficient spatial selection in 3D point cloud datasets consisting of thousands or millions of particles can be particularly challenging. We present two new techniques, TeddySelection and CloudLasso, that support the selection of subsets in large particle 3D datasets in an interactive and visually intuitive manner. Specifically, we describe how to spatially select a subset of a 3D particle cloud by simply encircling the target particles on screen using either the mouse or direct-touch input. Based on the drawn lasso, our techniques automatically determine a bounding selection surface around the encircled particles based on their density. This kind of selection technique can be applied to particle datasets in several application domains. TeddySelection and CloudLasso reduce, and in some cases even eliminate, the need for complex multi-step selection processes involving Boolean operations. This was confirmed in a formal, controlled user study in which we compared the more flexible CloudLasso technique to the standard cylinder-based selection technique. This study showed that the former is consistently more efficient than the latter; in several cases the CloudLasso selection time was half that of the corresponding cylinder-based selection.
In a variety of application areas, the use of simulation steering in decision making is limited at best. Research focusing on this problem suggests that most user interfaces are too complex for the end user. Our goal is to let users create and investigate multiple, alternative scenarios without the need for special simulation expertise. To simplify the specification of parameters, we move from a traditional manipulation of numbers to a sketch-based input approach. Users steer both numeric parameters and parameters with a spatial correspondence by sketching a change onto the rendering. Special visualizations provide immediate visual feedback on how the sketches are transformed into boundary conditions of the simulation models. Since uncertainty with respect to many intertwined parameters plays an important role in planning, we also allow the user to intuitively setup complete value ranges, which are then automatically transformed into ensemble simulations. The interface and the underlying system were developed in collaboration with experts in the field of flood management. The real-world data they have provided has allowed us to construct scenarios used to evaluate the system. These were presented to a variety of flood response personnel, and their feedback is discussed in detail in the paper. The interface was found to be intuitive and relevant, although a certain amount of training might be necessary.
The process of surface perception is complex and based on several influencing factors, e.g., shading, silhouettes, occluding contours, and top down cognition. The accuracy of surface perception can be measured and the influencing factors can be modified in order to decrease the error in perception. This paper presents a novel concept of how a perceptual evaluation of a visualization technique can contribute to its redesign with the aim of improving the match between the distal and the proximal stimulus. During analysis of data from previous perceptual studies, we observed that the slant of 3D surfaces visualized on 2D screens is systematically underestimated. The visible trends in the error allowed us to create a statistical model of the perceived surface slant. Based on this statistical model we obtained from user experiments, we derived a new shading model that uses adjusted surface normals and aims to reduce the error in slant perception. The result is a shape-enhancement of visualization which is driven by an experimentally-founded statistical model. To assess the efficiency of the statistical shading model, we repeated the evaluation experiment and confirmed that the error in perception was decreased. Results of both user experiments are publicly-available datasets.
The study of aerosol composition for air quality research involves the analysis of high-dimensional single particle mass spectrometry data. We describe, apply, and evaluate a novel interactive visual framework for dimensionality reduction of such data. Our framework is based on non-negative matrix factorization with specifically defined regularization terms that aid in resolving mass spectrum ambiguity. Thereby, visualization assumes a key role in providing insight into and allowing to actively control a heretofore elusive data processing step, and thus enabling rapid analysis meaningful to domain scientists. In extending existing black box schemes, we explore design choices for visualizing, interacting with, and steering the factorization process to produce physically meaningful results. A domain-expert evaluation of our system performed by the air quality research experts involved in this effort has shown that our method and prototype admits the finding of unambiguous and physically correct lower-dimensional basis transformations of mass spectrometry data at significantly increased speed and a higher degree of ease.
This paper presents the Element Visualizer (ElVis), a new, open-source scientific visualization system for use with highorder finite element solutions to PDEs in three dimensions. This system is designed to minimize visualization errors of these types of fields by querying the underlying finite element basis functions (e.g., high-order polynomials) directly, leading to pixel-exact representations of solutions and geometry. The system interacts with simulation data through runtime plugins, which only require users to implement a handful of operations fundamental to finite element solvers. The data in turn can be visualized through the use of cut surfaces, contours, isosurfaces, and volume rendering. These visualization algorithms are implemented using NVIDIA's OptiX GPU-based ray-tracing engine, which provides accelerated ray traversal of the high-order geometry, and CUDA, which allows for effective parallel evaluation of the visualization algorithms. The direct interface between ElVis and the underlying data differentiates it from existing visualization tools. Current tools assume the underlying data is composed of linear primitives; high-order data must be interpolated with linear functions as a result. In this work, examples drawn from aerodynamic simulations, high-order discontinuous Galerkin finite element solutions of aerodynamic flows in particular, will demonstrate the superiority of ElVis' pixel-exact approach when compared with traditional linear-interpolation methods. Such methods can introduce a number of inaccuracies in the resulting visualization, making it unclear if visual artifacts are genuine to the solution data or if these artifacts are the result of interpolation errors. Linear methods additionally cannot properly visualize curved geometries (elements or boundaries) which can greatly inhibit developers' debugging efforts. As we will show, pixel-exact visualization exhibits none of these issues, removing the visualization scheme as a source of uncertainty for engineers using ElVis.
We present KnotPad, an interactive paper-like system for visualizing and exploring mathematical knots; we exploit topological drawing and math-aware deformation methods in particular to enable and enrich our interactions with knot diagrams. Whereas most previous efforts typically employ physically based modeling to simulate the 3D dynamics of knots and ropes, our tool offers a Reidemeister move based interactive environment that is much closer to the topological problems being solved in knot theory, yet without interfering with the traditional advantages of paper-based analysis and manipulation of knot diagrams. Drawing knot diagrams with many crossings and producing their equivalent is quite challenging and error-prone. KnotPad can restrict user manipulations to the three types of Reidemeister moves, resulting in a more fluid yet mathematically correct user experience with knots. For our principal test case of mathematical knots, KnotPad permits us to draw and edit their diagrams empowered by a family of interactive techniques. Furthermore, we exploit supplementary interface elements to enrich the user experiences. For example, KnotPad allows one to pull and drag on knot diagrams to produce mathematically valid moves. Navigation enhancements in KnotPad provide still further improvement: by remembering and displaying the sequence of valid moves applied during the entire interaction, KnotPad allows a much cleaner exploratory interface for the user to analyze and study knot equivalence. All these methods combine to reveal the complex spatial relationships of knot diagrams with a mathematically true and rich user experience.
Geoscientific modeling and simulation helps to improve our understanding of the complex Earth system. During the modeling process, validation of the geoscientific model is an essential step. In validation, it is determined whether the model output shows sufficient agreement with observation data. Measures for this agreement are called goodness of fit. In the geosciences, analyzing the goodness of fit is challenging due to its manifold dependencies: 1) The goodness of fit depends on the model parameterization, whose precise values are not known. 2) The goodness of fit varies in space and time due to the spatio-temporal dimension of geoscientific models. 3) The significance of the goodness of fit is affected by resolution and preciseness of available observational data. 4) The correlation between goodness of fit and underlying modeled and observed values is ambiguous. In this paper, we introduce a visual analysis concept that targets these challenges in the validation of geoscientific models; specifically focusing on applications where observation data is sparse, unevenly distributed in space and time, and imprecise, which hinders a rigorous analytical approach. Our concept, developed in close cooperation with Earth system modelers, addresses the four challenges by four tailored visualization components. The tight linking of these components supports a twofold interactive drill-down in model parameter space and in the set of data samples, which facilitates the exploration of the numerous dependencies of the goodness of fit. We exemplify our visualization concept for geoscientific modeling of glacial isostatic adjustments in the last 100,000 years, validated against sea levels indicators; a prominent example for sparse and imprecise observation data. An initial use case and feedback from Earth system modelers indicate that our visualization concept is a valuable complement to the range of validation methods.
This paper introduces a new feature analysis and visualization method for multifield datasets. Our approach applies a surface-centric model to characterize salient features and form an effective, schematic representation of the data. We propose a simple, geometrically motivated, multifield feature definition. This definition relies on an iterative algorithm that applies existing theory of skeleton derivation to fuse the structures from the constitutive fields into a coherent data description, while addressing noise and spurious details. This paper also presents a new method for non-rigid surface registration between the surfaces of consecutive time steps. This matching is used in conjunction with clustering to discover the interaction patterns between the different fields and their evolution over time. We document the unified visual analysis achieved by our method in the context of several multifield problems from large-scale time-varying simulations.